Welcome to ASEED’s synthetic Nitrogen Fertilizer (N-fertilizer) FAQ. Here you can find all the information about why synthetic N-Fertilizer cannot be part of a fossil(fuel)free ecological agriculture, who are the big actors in the fertilizer businesses, and how we can embrace a relationship with the soil that is regenerative and life-sustaining. This FAQ is a constant work in progress, if you think there is something missing in this FAQ or something is still unclear feel free to contact us at info@aseed.net.
I. The Basics
Before we dive deeper into synthetic N-fertilizer we first want to share some basics about fertilizers and the nutrient cycling in the ecosystem: Nitrogen [N], phosphorus[P], and potassium [K – Kalium (Lat)] are the “Big 3” primary nutrients in commercial fertilizers. While nitrogen can be found gaseous (78%of the atmosphere consists of nitrogen (N2), dissolved in water and in solid inorganic or organic compounds, phosphorus can only be found in solid form as mineral or inorganic compounds. Ecosystems contain these and many other nutrients in a cycle. The roots absorb the nutrients, the plants grow, are consumed, or wilt, and through a cycle, the material eventually ends up in the ground again. With floods or harvests (and long transport routes) nutrients are removed from a system. Through various processes, these are replaced. The natural way is mineralization out of the soil and the nitrogen fixation from the air by legumes (plants that are able to fix nitrogen[N] out of the air, e.g. clover).
1. What is synthetic fertilizer and how is it different from other natural fertilizers?
While natural fertilizers don’t need to be synthesized in the lab, synthetic fertilizers are composed of inorganic compounds that are combined through a chemical process. Nitrogen can be produced synthetically, literally out of the air, but with a huge amount of energy cost (see Q.2+3, and the Haber-Bosch-Process). Potassium and Phosphor are being mined on a large scale and industrially processed for the fields. Instead of mineralization out of the bedrock (the rock under the soil), a huge amount of material is dug out, transported to chemical plants where the elements are split up and reassembled in a chemical process to make them more available to plants. The final product-synthetic fertilizer-often comes as a granulate or a liquid with different nutrient compositions.
The largest difference between synthetic and natural fertilizer is that the former needs a huge centralized production site, consumes a lot of energy and the final product is commercialized and sold to farmers. Synthetic fertilizer is presented by agri-industry as an efficient solution for farmers to aid soil infertility and to achieve higher yields, however, they rarely talk about long-term negative effects (see Q.4+6) and their main goal is to generate profit with their sales. Natural fertilizer, however, can be a more DIY, community-based, and decentralized alternative. Of course, you can have a huge biomass plant that collects a lot amount of biowaste and sells the compost eventually. But also small farmers all over the world can use environmentally appropriate techniques to fertilize their soil. More about natural ways to fertilize the soil can be found under Q5.
Alternative terms to synthetic nitrogen fertilizer you may have encountered are chemical fertilizer, inorganic fertilizer, artificial fertilizer. We chose to go with the term “synthetic” as it implies that the fertilizer was synthesized which emphasizes the GHG intensive production process (as described under Q3).
2. When and why did humans start using synthetic N-fertilizer?
At the beginning of the 20th-century scientists, Fritz Haber and Carl Bosch developed the so-called “Haber-Bosch” process, a very energy-intensive process to synthesize ammonia (NH3), which makes nitrogen available for plants. Initially, this process was used to produce explosives (ammonia is very reactive) for the First World War but in the 1960sthe process gained increasing popularity to produce large quantities of N-Fertilizer [1]. While in 1960 Global N-Fertilizer consumption was at 10.8 Mt N/year it has increased 8-fold (!!!) to 85.6 Mt N/year in 2000 [2]. The perceived advantage of N-fertilizer at that time was that it could produce a much larger quantity of food in a short time as the same (mono-)crop could be grown in the same location over and over again without the need to rotate it for with naturally nitrogen-fixing plants (how that works is explained in 3 & 4) [1]. This lead to skyrocketing yields of crops such as corn and today N-fertilizer allows for the industrial food system of monocultures to persist. The graph below illustrates how human population growth, predominantly in the Global North where SNF was more widely available at that time, was enabled through the invention of the Haber-Bosch process and the sharply increasing yields that SNF use on fields allowed for.

3. What do fossil fuels have to do with synthetic N-fertilizer?
Fossil fuels and GHG emissions are inherent to the production of SNF. Firstly, in the Haber-Bosch Process Hydrogen (H2) from fossil gas (CH4) is required to fix Nitrogen (N) to produce ammonia (NH3). Secondly, this process requires high pressure and very high temperatures of 400°C to 600°C. To achieve this, a lot of energy is needed and it is estimated that 3%- 5% percent of all natural gas produced and around 1%-2% percent of the global energy supply are used alone for ammonia production [3]. This means that the production of N-fertilizer is heavily dependent on fossil fuel extraction [2] and contributes to GHG emissions from burning them to produce high temperatures.
4. Isn’t synthetic N-fertilizer essential to grow enough food?
Nitrogen is an essential component for plant growth and if not enough of it is present it can limit the growth of a plant [4]. However, while the use of synthetic nitrogen fertilizer initially leads to higher yields, in the longterm it leads to losses of soil nutrients, which are essential for the long-term maintenance of soil fertility [5]. Eventually, it also causes the depletion of nitrogen as well as carbon which is usually naturally available in the soil [6]. This means a vicious cycle of natural soil fertility being depleted which is trying to be countered with application of even more synthetic nitrogen which only further depletes soil fertility in the longterm…and so on. In this way, over decades, our agricultural system has grown dependent on synthetic N-fertilizers tied to the perpetuation of the current trajectories of our industrial farming system [7]. However, feeding everyone on this planet is also a question of reducing (or ideally, eliminating!) food waste and changing Western diets. If these issues would be addressed, it is estimated that “affluent countries” (such as the USA and countries in Europe) could reduce their synthetic N-fertilizer consumption by 50% [4]. So in short, we actually don’t need SNF to grow enough food, we only need it if we want to continue on the destructive pathway of large scale monocultures, the use of fossil fuel-dependent machines, and unsustainable irrigation systems techniques which have only been supported through the use of SNF in the first place.
5. What are the alternatives to synthetic fertilizer?
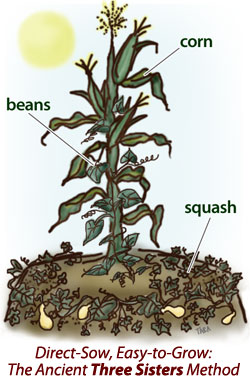
Image credit: University of Illinois Extension
In farming systems not relying on external inputs such as synthetic fertilizer, alternative ways are used to provide the plants with additional nutrients. Traditionally, compost from the previous harvest or manure from animals (that had eaten the plants on the field) was used and external inputs minimized. This allowed for the nutrient-cycle to be closed, as you can see in the picture in the beginning. Before synthetic fertilizers were introduced, another way to provide nitrogen to plants was planting nitrogen-fixing plants either by interplanting them with other crops or through crop rotation. Nitrogen-fixing plants such as clover, beans, peas, lentils, and lupines (leguminous plants) live in symbiosis with a bacteria in their roots called Rhizobium. These bacteria can fix nitrogen from the air and store it in the roots where it is then available for other plants. In Europe, crop rotation was practiced at least since medieval times and a commonly practiced system was the three-field system. One section was planted in the autumn with Rye or Winter wheat, the second section grew nitrogen-fixing crops such as peas, lentils, or beans, and the third field was left fallow. The following year this would be switched and so the section where the legumes had been growing provided lots of nitrogen for the cereal crops that deplete the soil of nitrogen. Before the Americas were colonized, similar practices could be found there as well, for example, the well-known system of the “Three Sisters”. This does not rely on crop rotation but interplanting corn, bean, and squash. Here, the beans provide the fixed nitrogen directly to the corn growing next to it. Crop rotation and interplanting used to be common methods for providing nitrogen naturally to plants and while the rise of synthetic fertilizer tried to make these systems obsolete, they are still practiced by ecological farmers and indigenous people today.
6. How does N-fertilizer influence the environment?
With the use of synthetic N-fertilizer humans have altered the natural nitrogen balance and disturbed the global nitrogen cycle which has serious consequences for our sensitive ecosystem (check out the graph below) [5]. When synthetic N-fertilizer is applied to the soil, plants absorb a part, but not all of it. Consequently, it instead ends up in the environment leading to various negative ecological consequences:
In the Soil: Nitrates leaching through the soil can cause the soil to become increasingly acidic and also depletes soil minerals. With rainfall, the nutrients were released from the soil into the water cycle. There, excessive input can have serious consequences for the ecosystem.
In the Water: It also leaches into the water bodies such as estuaries and coastal seas where the nutrients added through the nitrates cause extensive algae growth. This excessive algae growth is also referred to as ‘eutrophication’ which leads to oxygen depletion in the water posing serious problems for the survival of organisms such as fish and plankton to survive under these conditions [7]. In extreme (but by no means rare) cases this can lead to so-called ‘dead zones’, as we can find them for example at XYZ coast today, an area in the ocean that has become so oxygen-depleted through eutrophication that they cannot support any life anymore.
In The Atmosphere: In addition to this, increased input of synthetic N-fertilizer and to much manure to soil also increases the emission of Nitrous Oxide (N2O) from the soil [8]. N2O is a potent greenhouse gas and is a major contributor to ozone-layer depletion and around 60% of global N2O emissions come from agricultural soils [9].
7. Who is mainly involved in producing synthetic nitrogen fertilizer?
Below you can see the largest corporations that are involved in producing and selling N-fertilizer. With the free the soil campaign we want to focus on YARA, a Norwegian based company producing mainly N-fertilizer. More information about Yara you can find our in our brochure: “Yara, the company”.
The forth biggest ammonia producer is a dutch company. The production site in the Netherlands of YARA and OCI Nitrogen produce 5.7 Mt CO2eq per year. That is about 3% of the hole greenhouse gas emissions from the Netherlands. [12]
Production capacity of ammonia (N4), the most produced Nitrogen fertilizer.
Company | Production capacity | Headquarters location |
---|---|---|
C + F Industries | 9’900’000 t/a | USA |
Yara | 9’100’000 t/a | Norwegian |
Nutrient | 7’200’000 t/a | Canada |
OCI Nitrogen | 5’600’000 t/a | Netherlands |
OstChem | 3’700’000 t/a | Russia |
II. Intersections with Other Socio-Environmental Justice Issues
1. What does synthetic N-fertilizer have to do with our meat consumption and intensive mass animal farming?
If you look at the graph from Q1 again, you can notice that simultaneously with the increased use of synthetic N-fertilizer, meat consumption has also increased. And this is no coincidence – there is a direct connection between today’s ability for so many animals to be raised and the invention of synthetic N-fertilizer. Synthetic N-fertilizer has allowed for animal feed crops to be cultivated on a large scale with high yields in monocultures. This enabled humans to establish a system of mass animal farming which, in turn, has increased and is now sustaining our meat consumption. Of course, some animals are grass-fed but the majority is fed with cereal grains such as corn as well as soy [15] which are usually heavily fertilized. So without synthetic N-fertilizer humans would not be able to sustain a diet that is so intensive in meat products [14]. So what are the implications of this? It is widely recognized that eating meat is a highly inefficient way for humans to cover their protein needs, as the image below illustrates. Meat consumption has been discussed to be inefficient in using resources such as water, land, and fossil fuels [15] and, what is not often spoken about: meat as a protein source, in particular beef, means to inefficiently use synthetic N-fertilizer [15; 16]. The basic mechanism behind this is that “crops and grass need nitrogen to grow, while animals need proteins in which nitrogen is an essential element” (15, p135). However, only a small amount of the nitrogen is stored in the actual animal (what is later consumed as meat) as the majority of nitrogen is excremented. Essentially, through this a lot of the Nitrogen is lost and consuming a plant directly would mean to more efficiently use the Nitrogen available in the plant. Similar is true for other animal products such as milk, although the efficiency rate of converting protein (and the nitrogen contained in them) here is slightly higher. As we can see, changing our diets to more plant-based ones is essential if we want to reduce (and ideally eventually eliminate) the necessity for synthetic N-fertilizer and this is particular true for the Global North [16]. Abolishing intensive mass animal farming thus has the direct implication to drastically reduce the global synthetic N-fertilizer usage for growing animal feed crops.

production (Vaclav Smil, 2002)
2. What is the connection between nitrogen fertilizer and the nitrogen crisis in the Netherlands?
2017 the Netherlands used second most N-Fertilizer with 243 kg/ha in the world. The world average is 70 kg N/ha. [9] The Netherlands has a lot of farms between 30-100 ha and they are specialized for high yields and for that have high nutrient inputs. In addition to the synthetic fertilizer the manure of the many dairy farm gets also on the fields. This results in a total N-input of over 500 kg N/h
III. Further questions
These we want to try to answer in the future! If you want to help, please send a message to info[at]aseed[dot]net
X. How did those who cultivate land, farm before synthetic fertilizer was invented?
X. Why can it be difficult for farmers to just stop using SNF?
X. What short-term solutions does the industry propose?
X. What does synthetic N-fertilizer have to do with biofuels?
X. How does the nitrogen fertilizer business perpetuate neo-colonialism?
X. What is the connection between nitrogen fertilizer and other struggles such as socio-economic and gender-based issues?
IV. Sources
[1] Erisman, J. W., Sutton, M. A., Galloway, J., Klimont, Z., & Winiwarter, W. (2008). How a century of ammonia synthesis changed the world. Nature Geoscience, 1(10), 636-639.
[2] Crews, T. E., & Peoples, M. B. (2004). Legume versus fertilizer sources of nitrogen: ecological tradeoffs and human needs. Agriculture, ecosystems & environment, 102(3), 279-297.
[3] University of Tokyo: Press Release https://www.u-tokyo.ac.jp/focus/en/press/z0508_00041.html
[4] Chapin III, F. S., Vitousek, P. M., & Van Cleve, K. (1986). The nature of nutrient limitation in plant communities. The American Naturalist, 127(1), 48-58.
[5] Smil, V. (2002). Nitrogen and food production: proteins for human diets. AMBIO: A Journal of the Human Environment, 31(2), 126-131.
[6] Vitousek, P. M., Aber, J. D., Howarth, R. W., Likens, G. E., Matson, P. A., Schindler, D. W., … & Tilman, D. G. (1997). Human alteration of the global nitrogen cycle: sources and consequences. Ecological applications, 7(3), 737-750.[4.1] Mulvaney, R. L., Khan, S. A., & Ellsworth, T. R. (2009). Synthetic nitrogen fertilizers deplete soil nitrogen: a global dilemma for sustainable cereal production. Journal of environmental quality, 38(6), 2295-2314.
[7] Mancus, P. (2007). Nitrogen fertilizer dependency and its contradictions: a theoretical exploration of social‐ecological metabolism. Rural Sociology, 72(2), 269-288.
[8] Vitousek, P. M., Aber, J. D., Howarth, R. W., Likens, G. E., Matson, P. A., Schindler, D. W., … & Tilman, D. G. (1997). Human alteration of the global nitrogen cycle: sources and consequences. Ecological applications, 7(3), 737-750.
[9] Eichner, M. J. (1990). Nitrous Oxide Emissions from Fertilized Soils: Summary of Available Data. Journal of Environment Quality, 19(2), 272.
[10] Smith, K. A. (2017). Changing views of nitrous oxide emissions from agricultural soil: key controlling processes and assessment at different spatial scales. European Journal of Soil Science, 68(2), 137-155.
[11] https://ourworldindata.org/grapher/nitrogen-fertilizer-application-per-hectare-of-cropland?tab=table&time=earliest..latest
[12] https://www.pbl.nl/sites/default/files/downloads/pbl-2019-decarbonisation-options-for-the-dutch-fertiliser-industry_3657.pdf
[13] https://royalsociety.org/topics-policy/projects/low-carbon-energy-programme/green-ammonia/
[14] Aiking, H. (2011). Future protein supply. Trends in Food Science & Technology, 22(2-3), 112-120.
[15] Westhoek, H. J., Rood, G. A., van den Berg, M., Janse, J. H., Nijdam, D. S., Reudink, M. A., & Stehfest, E. E. (2011). The protein puzzle: the consumption and production of meat, dairy and fish in the European Union. European Journal of Nutrition & Food Safety, 123-144.
[16] Smil, V. (2002). Nitrogen and food production: proteins for human diets. AMBIO: A Journal of the Human Environment, 31(2), 126-131.